Imagine our Sun spewing out a flare 100 million times stronger than usual,
releasing the energy of 50 million trillion atomic bombs. The effect on our
planet would be catastrophic. Fortunately, what the Swift satellite has spotted
occurred a bit further away in a binary system called II Pegasi, some 135 light
years from Earth. Swift is designed to detect gamma-ray bursts — the most
powerful of all explosions — and this
flare was energetic enough to produce a false alarm for such a burst.
NOTE: The original entry here referenced the power of ‘fifty trillion’ atomic
bombs; the actual figure used by NASA was corrected, as above, to ‘fifty million
trillion.’ Thanks to Robin Goodfellow for catching the typo.
II Pegasi is an interesting system. The flare star is 0.8 times the mass of
the Sun; its companion is 0.4 solar masses, and the stars are separated by only
a few stellar radii. That produces fast rotation on both stars, and while II
Pegasi may be a billion years older than the Sun, the tight orbit has something
to do with the flare activity. “The tight binary orbit in II Pegasi acts as a
fountain of youth, enabling older stars to spin and flare as strongly as young
stars,” said Steve Drake of NASA Goddard, a co-author with Rachel Osten of an
upcoming Astrophysical Journal paper.
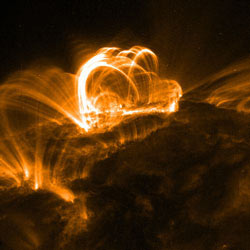
The cosmos is both a life-giving and a life-taking place. Some believe that
stellar outbursts like this one may be a factor in conditioning interstellar
dust that will later form into planets, immense violence that eventually
produces life. But such a flare could be a civilization-ender if it occurred on
a star like ours. The x-ray outpouring would produce climate change and,
doubtless, mass extinction. X-ray emissions from II Pegasi lasted for several
hours; a normal solar flare produces emissions that may last a few minutes.
Image: A typical solar flare from our sun, from September
2005, captured in the X-ray waveband by NASA’s TRACE satellite. Note the bright
magnetic loops of matter. The twisting and reconnecting of these loops initiate
the flare. NASA’s Swift satellite detected a similar flare from a star system
called II Pegasi 135 light-years from Earth… except it was one hundred million
times more energetic than the sun’s typical solar flare. Had it been from our
sun, it would have triggered a mass extinction on Earth. The II Pegasi flare was
too distant (fortunately) to image in detail. Credit: NASA/LMSAL.
Flares aren’t on the agenda, but extinction events of a more localized sort
will be discussed at the 2007
Planetary Defense Conference, to be held next March 5-8 at George Washington
University in Washington, DC. The objective of the conference is to create a
white paper that analyzes the current state of the art in detection and tracking
of near Earth objects (NEOs), and the methods we might use to defend ourselves
against them. Registration opens in December; let’s hope the conference gets
plenty of press coverage.
And finally, a 48-minute documentary
with the same name — “Planetary Defense” — is now being produced to depict the
various efforts at detection and deflection of asteroids and comets with
Earth-crossing orbits. Numerous experts in the field are said to be involved,
along with science fiction legend Arthur C. Clarke, who began his 1973 novel
Rendezvous with Rama with a major asteroid impact (on a September 11,
no less, though one set 75 years in the book’s future). Project Spaceguard is
the result, a determined response to incoming objects that, we must hope, will
one day become reality in the form of a robust space-based infrastructure
throughout our Solar System.